LONGITUDINAL SOUND WAVES
Sound is a longitudinal wave that is created by a vibrating object, such as a guitar string, the human vocal cords, or the diaphragm of a loudspeaker. Moreover, sound can be created or transmitted only in a medium, such as a gas, liquid, or solid. As we will see, the particles of the medium must be present for the disturbance of the wave to move from place to place. Sound cannot exist in a vacuum.
To see how sound waves are produced and why they are longitudinal, consider the vibrating diaphragm of a loudspeaker. When the diaphragm moves outward, it compresses the air directly in front of it, as in Figure 16.12a. This compression causes the air pressure to rise slightly. The region of increased pressure is called a condensation, and it travels away from the speaker at the speed of sound. The condensation is analogous to the compressed region of coils in a longitudinal wave on a Slinky, which is included in Figure 16.12a for comparison. After producing a condensation, the diaphragm reverses its motion and moves inward, as in part b of the drawing. The inward motion produces a region known as a rarefaction, where the air pressure is slightly less than normal. The rarefaction is similar to the stretched region of coils in a longitudinal Slinky wave. Following immediately behind the condensation, the rarefaction also travels away from the speaker at the speed of sound. Figure 16.13 further emphasizes the similarity between a sound wave and a longitudinal Slinky wave. As the wave passes, the colored dots attached both to the Slinky and to an air molecule execute simple harmonic motion about their undisturbed positions. The colored arrows on either side of the dots indicate that the simple harmonic motion occurs parallel to the line of travel. The drawing also shows that the wavelength l is the distance between the centers of two successive condensations; l is also the distance between the centers of two successive rarefactions.
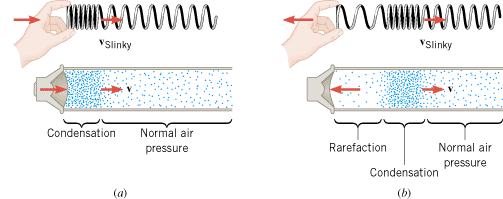 | Figure 16.12
(a) When the speaker diaphragm moves outward, it creates a condensation. (b) When the diaphragm moves inward, it creates a rarefaction. The condensation and rarefaction on the Slinky are included for comparison. In reality, the velocity of the wave on the Slinky v
Slinky is much smaller than the velocity of sound in air v. For simplicity, the two waves are shown here to have the same velocity. |
|
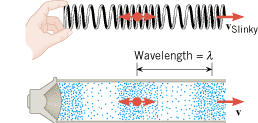 | Figure 16.13
Both the wave on the Slinky and the sound wave are longitudinal. The colored dots attached to the Slinky and to an air molecule vibrate back and forth parallel to the line of travel of the wave. |
|
Figure 16.14 illustrates a sound wave spreading out in space after being produced by a loudspeaker. When the condensations and rarefactions arrive at the ear, they force the eardrum to vibrate at the same frequency as the speaker diaphragm. The vibratory motion of the eardrum is interpreted by the brain as sound. It should be emphasized that sound is not a mass movement of air, like the wind. As the condensations and rarefactions of the sound wave travel outward from the vibrating diaphragm in Figure 16.14, the individual air molecules are not carried along with the wave. Rather, each molecule executes simple harmonic motion about a fixed location. In doing so, one molecule collides with its neighbor and passes the condensations and rarefactions forward. The neighbor, in turn, repeats the process.
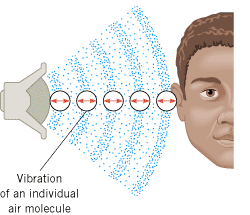 | Figure 16.14
Condensations and rarefactions travel from the speaker to the listener, but the individual air molecules do not move with the wave. A given molecule vibrates back and forth about a fixed location. |
|
THE FREQUENCY OF A SOUND WAVE
Each cycle of a sound wave includes one condensation and one rarefaction, and the frequency is the number of cycles per second that passes by a given location. For example, if the diaphragm of a speaker vibrates back and forth in simple harmonic motion at a frequency of 1000 Hz, then 1000 condensations, each followed by a rarefaction, are generated every second, thus forming a sound wave whose frequency is also 1000 Hz. A sound with a single frequency is called a pure tone. Experiments have shown that a healthy young person hears all sound frequencies from approximately 20 to 20 000 Hz (20 kHz). The ability to hear the high frequencies decreases with age, however, and a normal middle-aged adult hears frequencies only up to 12–14 kHz.
Pure tones are used in push-button telephones, such as that shown in Figure 16.15. These phones simultaneously produce two pure tones when each button is pressed, a different pair of tones for each different button. The tones are transmitted electronically to the central telephone office, where they activate switching circuits that complete the call. For example, the drawing indicates that pressing the “5” button produces pure tones of 770 and 1336 Hz simultaneously, while the “9” button generates tones of 852 and 1477 Hz.
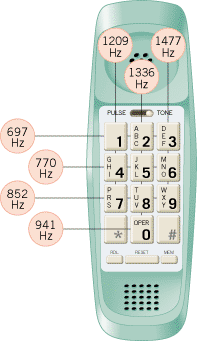 | Figure 16.15
A push-button telephone and a schematic showing the two pure tones produced when each button is pressed. |
|
Sound can be generated whose frequency lies below 20 Hz or above 20 kHz, although humans normally do not hear it. Sound waves with frequencies below 20 Hz are said to be infrasonic, while those with frequencies above 20 kHz are referred to as ultrasonic. Rhinoceroses use infrasonic frequencies as low as 5 Hz to call one another (Figure 16.16), while bats use ultrasonic frequencies up to 100 kHz for locating their food sources and navigating (Figure 16.17).
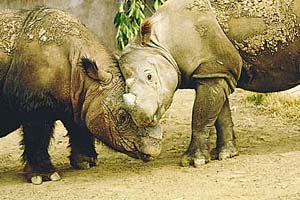 | Figure 16.16
Rhinoceroses call to one another using infrasonic sound waves. (Photo by Ron Garrison, courtesy Zoological Society of San Diego.) |
|
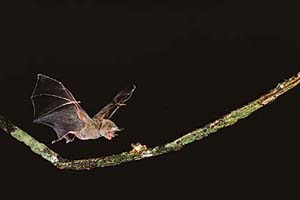 | Figure 16.17
Bats use ultrasonic sound waves for navigating and locating food sources. (© Merlin D. Tuttle/Bat Conservation International/Photo Researchers) |
|
Frequency is an objective property of a sound wave because frequency can be measured with an electronic frequency counter. A listener’s perception of frequency, however, is subjective. The brain interprets the frequency detected by the ear primarily in terms of the subjective quality called pitch. A pure tone with a large (high) frequency is interpreted as a high-pitched sound, while a pure tone with a small (low) frequency is interpreted as a low-pitched sound. A piccolo produces high-pitched sounds, and a tuba produces low-pitched sounds.
THE PRESSURE AMPLITUDE OF A SOUND WAVE
Figure 16.18 illustrates a pure-tone sound wave traveling in a tube. Attached to the tube is a series of gauges that indicate the pressure variations along the wave. The graph shows that the air pressure varies sinusoidally along the length of the tube. Although this graph has the appearance of a transverse wave, remember that the sound itself is a longitudinal wave. The graph also shows the pressure amplitude of the wave, which is the magnitude of the maximum change in pressure, measured relative to the undisturbed or atmospheric pressure. The pressure fluctuations in a sound wave are normally very small. For instance, in a typical conversation between two people the pressure amplitude is about 3
×
102 Pa, certainly a small amount compared with the atmospheric pressure of 1.01
×
10+5 Pa. The ear is remarkable in being able to detect such small changes.
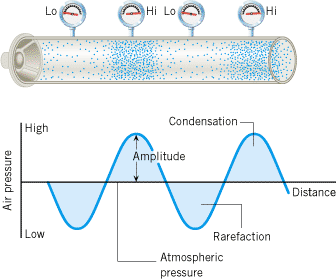 | Figure 16.18
A sound wave is a series of alternating condensations and rarefactions. The graph shows that the condensations are regions of higher than normal air pressure, and the rarefactions are regions of lower than normal air pressure. |
|
Loudness is an attribute of sound that depends primarily on the amplitude of the wave: the larger the amplitude, the louder the sound. The pressure amplitude is an objective property of a sound wave, since it can be measured. Loudness, on the other hand, is subjective. Each individual determines what is loud, depending on the acuteness of his or her hearing.
 |
Copyright © 2000-2003 by John Wiley & Sons, Inc. or related companies. All rights reserved. |